Research areas
The research areas of the working group "Batteries - Calorimetry and Safety" focus on the investigation of the safety of lithium-ion cells as well as post-lithium cells. In particular, calorimetric methods are used to take an in-depth look at a wide range of aspects of safety in battery cells.
Starting with our own production of small cells, the thermal properties of these are investigated. The research focuses in particular on comprehensive aging tests, which include both calendar and cyclic aging. With the help of calorimeters, detailed information on heat generation under normal and abuse scenarios will be performed. In addition, thermal propagation at the cell and pack level will be investigated. The research areas are complemented by electrochemical impedance spectroscopy and modeling approaches.
Cell construction
Construction of small cells and investigation of the thermal properties of cell components.
Button cells are built using a press located in a glovebox under an inert argon atmosphere. These are mostly half cells in which Li metal is used as one electrode and the material to be tested as the other electrode. Both are separated by a glass fiber separator impregnated with electrolyte. Prior to this, the thermal properties of each cell component are determined using the C80 3D Calvet calorimeter or other thermophysical methods available in the Thermophysics and Thermodynamics Group. The finished button cells are first formed in temperature chambers at constant temperature, i.e., cycled at low current rates to allow the formation of the SEI (Solid Electrolyte Interface) layer to protect the anode, which is critical for cell lifetime. This is followed by electrochemical characterization.
Aging tests

Comprehensive aging tests are performed at the cell level. First, fresh cells are stored in temperature chambers at different temperatures and charge states and then characterized at specified time intervals to investigate the influence of this storage (calendar aging) on cell performance. Second, similar cells are aged at different charge/discharge rates or with different load profiles (full cycles, partial cycles, drive cycles) in temperature chambers (cyclic aging) and also comprehensively characterized electrochemically and thermally at specific times. In addition, fresh cells, aged cells and cells after a thermal runaway can be opened in a glovebox and the components and active materials can be characterized in detail in a post-mortem analysis using physicochemical and materials science analysis methods.
Heat generation during normal use
The thermal impedance of the cells plays an important role, since this variable influences the heat flows and thus also the heat and temperature distribution in an individual cell and also in a complete battery system. This is determined by the thermophysical transport quantities such as thermal and thermal diffusivity, as well as by the heat capacity. In addition, thermal transfer resistances are an important factor influencing the spatial and temporal behavior of temperature fields in the cell, which in turn are also influenced by aging processes in the individual cell. Calorimetry is used to quantitatively determine the occurring thermal effects very precisely and to relate them to the aging state of the cells and the electrolyte reactions.
Using accelerating rate calorimeters, as well as electrochemical impedance spectroscopy (EIS), current interruption methods (CIT) and potentiometric entropy measurement, the following electrochemical, thermodynamic and thermal parameters can be measured for larger pouch and prismatic automotive cells:
- Capacity
- Cycle efficiency
- Specific heat capacity
- Thermal conductivity
- Heat dissipation
- Spatially/time resolved temperature/heat evolution
- Generated heat (Irreversible/Reversible fractions)
By dividing the generated heat into reversible fractions, related to the entropy of the cell reaction, and irreversible fractions, related to the internal resistance of the cell, the characterization of the calendrical and cyclic aging behavior is enabled.
Heat, gas and pressure generation with electrical, mechanical, thermal abuse.
Non-regular use and simulated accident studies are also performed in the Accelerating Rate Calorimeters. Exothermic, out-of-control reactions leading to thermal runaway can be quantitatively measured and analyzed for complete cells. Thus, the change in hazard potential of the cells with increasing degree of aging can also be quantitatively evaluated. The following safety tests are possible:
(a) Electrical tests: internal/external short circuit, overcharge, deep discharge.
In the ARC, the temperature rise can be measured by applying an external short circuit or during an internal short circuit, which can be caused, for example, by a production defect. The cell can also be overcharged or overdischarged, resulting in different failure modes.
b) Mechanical tests: Nail test, deformation test
A system controlled by a stepper motor allows the cell to be pierced with a pointed nail or deformed with a blunt test piece. The advantage of testing in a calorimeter over testing in a standard test fixture is that it is not only a pass/fail test to qualify cells, but valuable quantitative data is also obtained in the process.
c) Thermal tests: ramp heating test, heat-wait-seek test
For the ramp heating test, cells are heated at a constant heating rate until thermal runaway occurs. More detailed information is provided by the Heat-Wait-Seek (HWS) test. This begins by heating the sample in small steps and at the end of each step, the system "waits" to see if the cell generates heat that results in a measurable temperature rise ("seek" step). Once self-heating of the cell is detected, the system switches to quasiadiabatic mode so that the temperature of the calorimeter immediately follows the surface temperature of the cell (no more heat is exchanged with the environment). Thus, the cell heats up independently more and more until thermal runaway occurs, or the reactants are completely used up by the exothermic reactions, so that the reaction comes to a standstill and the next heating step follows.
Only the Accelerating Rate Calorimeter can replicate the Thermal Runaway and all the exothermic reactions that precede it, helping to analyze the stepwise individual processes, such as SEI decomposition, gas formation and the subsequent reactions during the Thermal Runaway, and to determine their activation energies.
The external pressure can be measured using a gas-tight cylinder in the calorimeter chamber to detect the opening (venting) of the cells. For internal pressure measurements, a capillary connected to a pressure cell is inserted directly into the cell and the pressure is recorded during the test.
Comparison of temperature-time plots for 18650 cells with different cathode materials during the HWS test in an ARC. | X-ray tomography image of an 18650 cell showing the position of the capillary and the pressure-temperature curves for the external and internal pressure measurements during the thermal abuse test in an ARC. |
As a result of the studies, quantitative and system relevant data for the temperature, heat and pressure evolution of the LIB will be provided. These data will then be used by research and industry partners for the design of thermal management and safety systems.
Thermal propagation tests at small pack level

For a comprehensive consideration of the safety aspects in lithium-ion cells, an investigation on pack level is necessary. With the calorimeters available at IAM-AWP, it is also possible to investigate the thermal behavior in small packs. In particular, additional components, such as heat shields, can be investigated with respect to their influence on thermal propagation. Ideally, these should protect surrounding cells from a thermal runaway or at least delay its onset by 5 to 10 minutes.
Electrochemical impedance spectroscopy and modeling
Electrochemical impedance spectroscopy (EIS) can be used to determine characteristic parameters of battery cells based on the relationship between current and voltage. Among other things, the internal resistance and the aging condition can be investigated. The data obtained serve as parameters for the modeling of battery systems.
POLiS
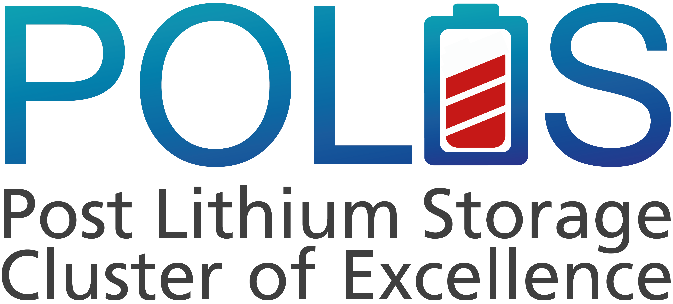
Thermal and detailed safety-related studies on the small-scale level (coin cells) are necessary prior to scaling up in order to achieve a deep understanding of the underlying reaction mechanisms and the processes of heat generation and dissipation, which are currently not known for the post-Li cells. Therefore, thermal stability data are acquired from anodes, cathodes, and electrolytes for post-Li cells. Furthermore, thermal effects due to chemical interactions will be measured as the main important part of this safety project. Heat sources will be identified which contribute to the electrodes during the charge and discharge cycling processes. Finally, the exothermic reactions will be identified according to their sequence of occurrence in the safety tests. This holistic safety assessment allows the identification of guiding principles for the safe combination of materials and for safe cell operation, which is a prerequisite for scaling up and market acceptance of new battery technologies. The expected outcome is to develop a thorough understanding of thermal behaviour and safety issues along the complete chain from active materials to complete cells.
Gas analysis
Under abuse conditions, Li-ion batteries undergo an exothermal state accompanied by gas evolution, which can result in a severe condition, the so-called thermal runaway. Gas analysis techniques are used to identify and quantify gaseous species emitted from batteries. Gas Chromatography – Mass Spectrometry (GC-MS) and online MS are good candidates for such an analysis. The conjunction of MS with GC in GC-MS provides a powerful technique and preferred to analyze small and volatile molecules, where the GC is critical to obtain a good separation of gas components, and the MS is responsible to distinguish fragmentations depending on the molecular structure of input compounds. Also, online MS is a technique that can help to understand and distinguish between different gas evolution events occurring in the batteries, sequentially, under the abuse conditions.
By the gas analysis, a mixture of gas species will be detected. Combining the information obtained about the evolved gas species with the knowledge of battery structure, chemistry, and working conditions (temperature, state of charge, etc.), internal reactions of battery can be understood and evaluated. As an example, it is often reported that at 100 – 120 °C, the Li-ion batteries experience solid electrolyte interface (SEI) decomposition which leads to production of ethylene (C2H4), carbon dioxide (CO2) and oxygen (O2).
Combining gas analysis with other characterization methods such as SEM, XRD, ICP-OES, the failure mechanisms of battery under abuse conditions (thermal, electrochemical, and mechanical) can be further explored, which can be therefore beneficial to improve battery state monitoring and promote design for battery safety
Gasanalyse
Unter Missbrauchsbedingungen kommt es bei Lithium-Ionen-Batterien zu einem exothermen Zustand, der mit einer Gasentwicklung einhergeht, die zu einem schwerwiegenden Zustand, dem so genannten thermischen Durchgehen, führen kann. Gasanalysetechniken werden zur Identifizierung und Quantifizierung der von den Batterien abgegebenen Gase eingesetzt. Gaschromatographie-Massenspektrometrie (GC-MS) und Online-MS sind gute Kandidaten für eine solche Analyse. Die Verbindung von MS mit GC in GC-MS bietet eine leistungsstarke Technik und wird bevorzugt für die Analyse kleiner und flüchtiger Moleküle eingesetzt, wobei die GC für eine gute Trennung der Gaskomponenten entscheidend ist und die MS für die Unterscheidung der Fragmentierungen in Abhängigkeit von der Molekularstruktur der Eingangsverbindungen verantwortlich ist. Außerdem ist die Online-MS eine Technik, die helfen kann, verschiedene Gasentwicklungsereignisse zu verstehen und zu unterscheiden, die in den Batterien nacheinander unter den Missbrauchsbedingungen auftreten.
Bei der Gasanalyse wird eine Mischung von Gasspezies festgestellt. Kombiniert man die Informationen über die entstandenen Gase mit der Kenntnis der Batteriestruktur, der Chemie und der Betriebsbedingungen (Temperatur, Ladezustand usw.), lassen sich die internen Reaktionen der Batterie verstehen und bewerten. So wird beispielsweise häufig berichtet, dass bei 100-120 °C in Li-Ionen-Batterien eine Zersetzung der Festelektrolyt-Grenzfläche (SEI) stattfindet, die zur Bildung von Ethylen (C2H4), Kohlendioxid (CO2) und Sauerstoff (O2) führt.
Durch die Kombination der Gasanalyse mit anderen Charakterisierungsmethoden wie SEM, XRD und ICP-OES können die Versagensmechanismen von Batterien unter missbräuchlichen Bedingungen (thermisch, elektrochemisch und mechanisch) weiter erforscht werden, was zur Verbesserung der Überwachung des Batteriezustands und zur Förderung des Designs für die Batteriesicherheit von Nutzen sein kann.